
The editing could serve to protect double-stranded RNAs from immune attack.
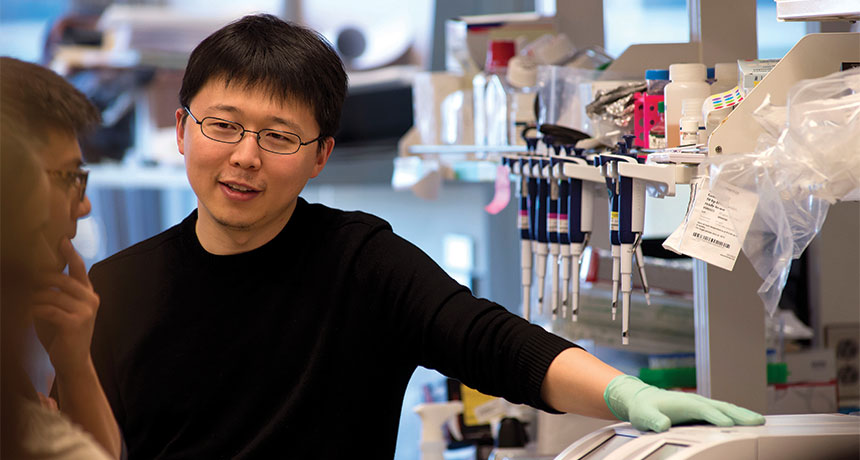
She says that no one has found a specific role for the changes made to non-protein-coding RNAs, which account for the majority of edited molecules. The editing might serve a regulatory function, but most adult tissues don’t produce the high levels of the proteins required for the editing to occur.īrenda Bass, a biochemist at the University of Utah in Salt Lake City, was among the first to identify ADARs in frog embryos. Some have speculated that the ADAR proteins evolved as a defence against viruses, but many viruses with double-stranded RNA are unaffected by the enzymes. The editors work only on double-stranded RNAs, which sometimes show up in the cell as regulatory elements-or as viruses. Scientists have struggled over the past three decades to understand what exactly RNA editing accomplishes. A handful of other RNA-editing enzymes surfaced around the same time. Although not one of the canonical RNA bases, inosine is read by the cell’s protein-translation machinery as the familiar guanosine. These proteins bind to RNAs and alter their sequence by changing a familiar base known as adenosine into a molecule called inosine. The findings were controversial until scientists uncovered a family of enzymes called adenosine deaminases acting on RNA (ADARs). But in the 1980s, a handful of labs noticed that some mRNA transcripts contained altered or extra letters that were not encoded in the DNA. A role for RNAĪ foundational tenet in molecular genetics-its central dogma-was that cellular machinery faithfully transcribes genetic information from a double-stranded DNA template into a single-stranded RNA messenger, which is then translated into a protein. “It really opens a world we haven’t seen before,” Stafforst says. Still, researchers hope that new technologies, such as protein engineering, and improved methods for delivering RNA to cells can help to overcome these limitations. Several hurdles remain: current technologies can alter RNA sequences in only a few limited ways, and getting the system to work as intended in the human body will prove challenging. And although RNA-based drugs have had difficulty reaching the market owing to challenges in delivery and tolerance, some regulatory approvals in the past few years might help to pave the way for RNA-editing therapies. A handful of start-up companies are beginning to use RNA-editing systems to develop potential treatments for everything from genetic diseases such as muscular dystrophy to temporary maladies such as acute pain.

In 2019, researchers published more than 400 papers on the topic, according to data from Scopus, an abstract and citation database. Because cells quickly degrade unused RNAs, any errors introduced by a therapy would be washed out, rather than staying with a person forever.Įxcitement over RNA editing is finally catching on. RNA editing, by contrast, could allow clinicians to make temporary fixes that eliminate mutations in proteins, halt their production or change the way that they work in specific organs and tissues. Researchers have documented ways that Cas9, one of the enzymes used in CRISPR gene editing, could trigger immune responses, or cause accidental changes to the genome that would be permanent. They asked, “Why do we need this when there’s DNA editing?”īut CRISPR editing-at least as a therapeutic technique in people-has turned out to be more difficult than initially thought. With CRISPR sucking up all the attention, Stafforst says, people reacted to his paper with indifference. Since then, CRISPR has become a fixture in the laboratory and has spawned a number of companies aimed at using the technology to develop drugs and treatments. His finding was overshadowed by the discovery a few months earlier that the DNA-editing tool CRISPR–Cas9 could be used to permanently alter the genome.

But Stafforst had a lot of trouble getting the discovery published-it was simply not interesting any more. The process could theoretically serve to treat numerous diseases, both ones with genetic underpinnings and those that would benefit from a change in the amount or type of a protein being produced. In essence, they could rewrite the genome’s instructions en route to making proteins. In 2012, his team at the University of Tübingen in Germany discovered that by linking enzymes to engineered strands of RNA, they could change the sequences of messenger RNA molecules in cells. Thorsten Stafforst found his big break at the worst possible time.
